Stereolithography (SLA) 3D printing has become vastly popular for its ability to produce high-accuracy, isotropic, and watertight prototypes and parts in a range of advanced materials with fine features and smooth surface finish.
In this comprehensive guide, learn how SLA printing technologies work, why thousands of professionals use this process today, and what you need to know to explore how this 3D printing process can benefit your work.
Introduction
Advancements in 3D printing continue to change the way businesses approach prototyping and production. As the technology becomes more accessible and affordable and hardware and materials advance to match market opportunities and demands, designers, engineers, and beyond are integrating 3D printing into workflows across development cycles.
Across industries, 3D printing is helping professionals cut outsourcing costs, iterate faster, optimize production processes, and even unlock entirely new business models.
Stereolithography 3D printing in particular has undergone significant changes. Traditionally, resin 3D printers have been monolithic and cost-prohibitive, requiring skilled technicians and costly service contracts. Today, small-format desktop printers produce industrial-quality output, at substantially more affordable price points and with unmatched versatility.
What is Stereolithography 3D Printing?
Stereolithography belongs to a family of additive manufacturing technologies known as vat photopolymerization, commonly known as resin 3D printing. These machines are all built around the same principle, using a light source—a laser or projector—to cure liquid resin into hardened plastic. The main physical differentiation lies in the arrangement of the core components, such as the light source, the build platform, and the resin tank.
SLA 3D printers use light-reactive thermoset materials called “resin.” When SLA resins are exposed to certain wavelengths of light, short molecular chains join together, polymerizing monomers and oligomers into solidified rigid or flexible geometries.
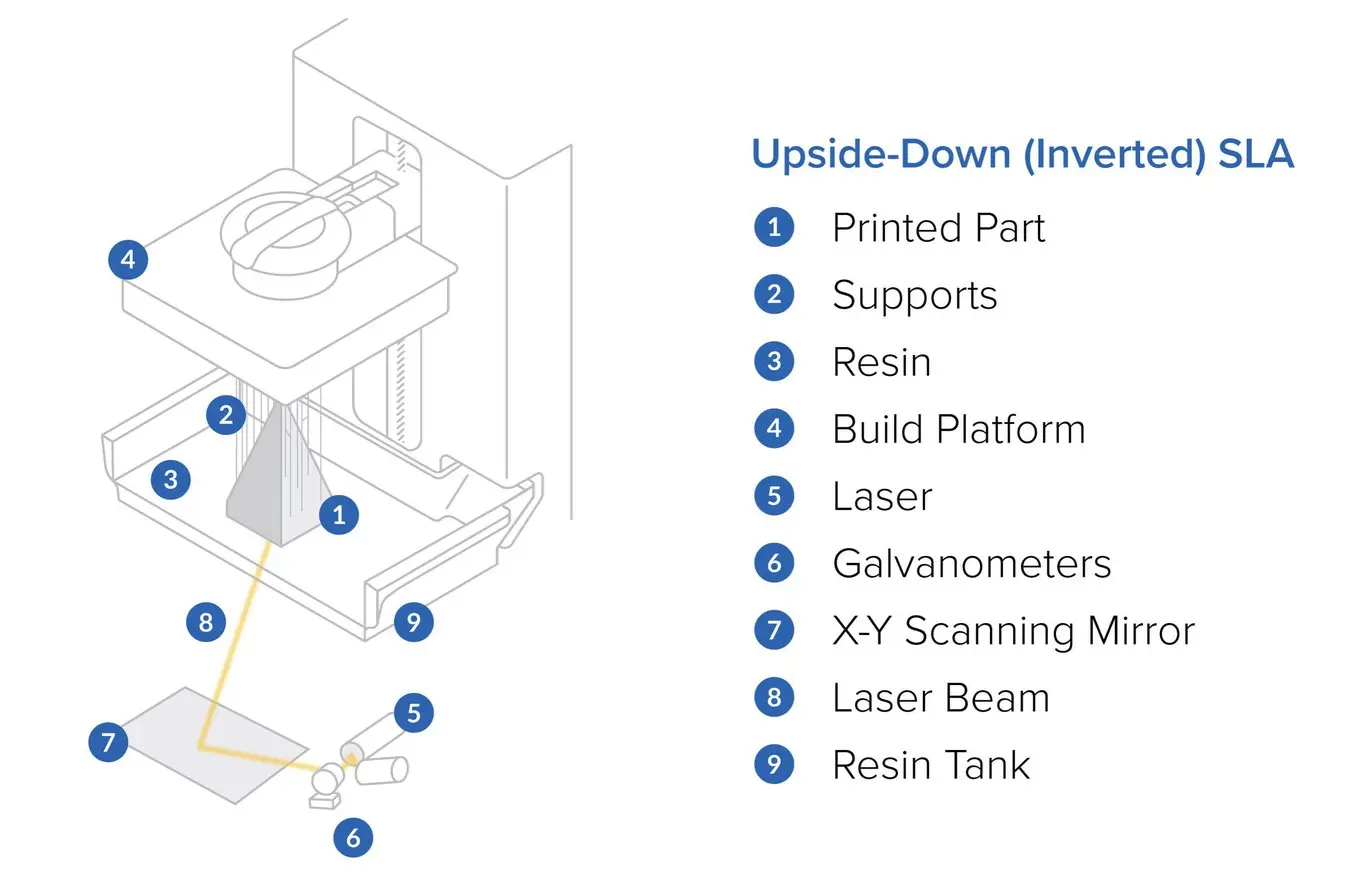
SLA parts have the highest resolution and accuracy, the sharpest details, and the smoothest surface finishes of all 3D printing technologies, but the main benefit of the stereolithography lies in its versatility.
Material manufacturers have created innovative SLA resin formulations with a wide range of optical, mechanical, and thermal properties to match those of standard, engineering, and industrial thermoplastics.
The SLA 3D Printing Workflow
See how to go from design to 3D print with the Form 3 SLA 3D printer. This 5-minute video covers the basics of how to use the Form 3, from the software and materials to printing and post-processing.
1. Design
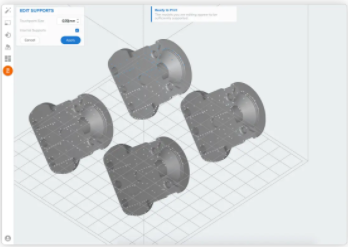
Use any CAD software or 3D scan data to design your model, and export it in a 3D printable file format (STL or OBJ). Each SLA printer includes software to specify printing settings and slice the digital model into layers for printing. Once setup is complete, the print preparation software sends the instructions to the printer via a wireless or cable connection.
More advanced users may consider specifically designing for SLA, or taking steps like hollowing parts to conserve material.
2. Print
After a quick confirmation of the correct setup, the printing process begins and the machine can run unattended until the print is complete. In printers with a cartridge system, the material is automatically refilled by the machine.
3. Post-Process
Once the printing is completed, parts require rinsing in isopropyl alcohol (IPA) to remove any uncured resin from their surface. After rinsed parts dry, some materials require post-curing, a process which helps parts to reach their highest possible strength and stability. Finally, remove supports from the parts and sand the remaining support marks for a clean finish. SLA parts can be easily machined, primed, painted, and assembled for specific applications or finishes.
Post-curing is particularly important for functional resins for engineering, and mandatory for some dentistry and jewelry materials and applications.
A Brief History of Stereolithography
The SLA 3D printing process first appeared in the early 1970s, when Japanese researcher Dr. Hideo Kodama invented the modern layered approach to stereolithography, using ultraviolet light to cure photosensitive polymers. The term stereolithography was coined by Charles (Chuck) W. Hull, who patented the technology in 1986 and founded the company 3D Systems to commercialize it. Hull described the method as creating 3D objects by successively “printing” thin layers of a material curable by ultraviolet light.
SLA 3D printing, however, was not the first 3D printing technology to gain widespread popularity. As patents began to expire at the end of the 2000s, the introduction of small format, desktop 3D printing widened access to additive manufacturing, with fused deposition modeling (FDM) first gaining adoption in desktop platforms.
While this affordable extrusion-based technology sparked the first wave of wide adoption and awareness of 3D printing, FDM machines did not satisfy the spectrum of professional needs—repeatable, high-precision results are crucial for professional applications, as are biocompatible materials in the dental industry and the ability to create fine features for industries like jewelry and applications like millifluidics.
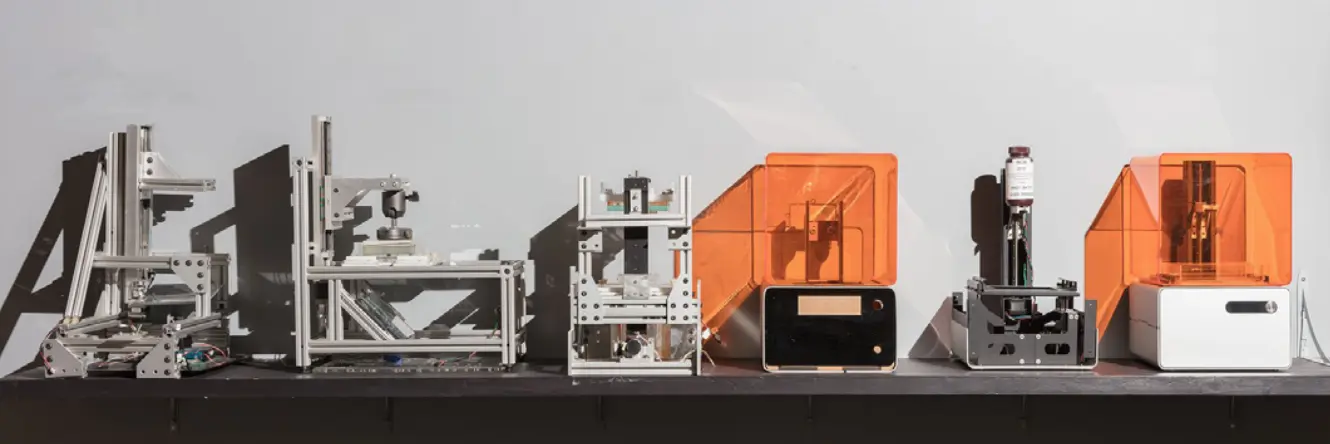
Desktop SLA Printers Disrupt the Market
SLA printing soon followed FDM to the desktop, when Formlabs adapted the technology in 2011. Small format desktop SLA printers brought the promise of high resolution 3D printing—previously limited to monolithic industrial systems—in a much smaller and more affordable setup with a wide range of print materials. These capabilities expanded access to 3D printing for a variety of custom and high precision applications across disciplines, including engineering, product design, and manufacturing, as well as dental, jewelry, and other industries.
In 2015, Formlabs released its next-generation SLA 3D printer, the Form 2, which became the industry-leading desktop 3D printer, with parts printed in the field ranging from affordable custom prosthetics to a customizable line of razor handles.
The Form 2 reset the conversation for SLA 3D printing, popularizing a “distributed” model of production, where companies can scale output incrementally, adding more small format printers as demand increases with the flexibility to print in different materials on each printer. The maturity of materials over time has only increased the usage of this model, as more advanced resins have unlocked applications beyond prototyping and into production and end-use parts across industries.
In 2019, Formlabs made another step change in the industry with the launch of the Form 3 and Form 3L, two new hardware products that set a new standard for SLA with systems built on a completely new print process.
The Next Chapter of SLA: Low Force Stereolithography 3D Printing
Low Force Stereolithography (LFS) technology is the next phase in SLA 3D printing, meeting the demands on today’s market for scalable, reliable, industrial-quality 3D printing.
This advanced form of SLA 3D printing drastically reduces the forces exerted on parts during the print process, using a flexible tank and linear illumination to deliver incredible surface quality and print accuracy. Lower print forces allow for light-touch support structures that tear away with ease, and the process opens up a wide range of possibilities for future development of advanced, production-ready materials.
Inverted SLA printing introduces peel forces that affect the print as it separates from the surface of the tank, so the build volume is limited and sturdy support structures are required. The Formlabs Form 2 is heavily calibrated to account for the forces of the peel process and produce high quality parts. Learn more about the differences between the Form 2 and Form 3, which uses LFS technology.
LFS 3D printing drastically reduces the forces exerted on parts during the print process, using a flexible tank and linear illumination to deliver incredible surface quality and print accuracy. Learn more about how LFS works in this deep dive video.
Why Choose SLA 3D Printing?
Engineers, designers, manufacturers, and more choose SLA 3D printing for its fine features, smooth surface finish, ultimate part precision and accuracy, and mechanical attributes like isotropy, watertightness, and material versatility.
Isotropy
Because 3D printing creates parts one layer at a time, completed prints may have variations in strength based on orientation of the part relative to the printing process, with different properties in X, Y, and Z axes.
Extrusion-based 3D printing processes like fused deposition modeling (FDM) are known for being anisotropic due to layer-to-layer differences created by the print process. This anisotropy limits the usefulness of FDM for certain applications or requires more adjustments on the part geometry side to compensate for it.
Read our in-depth guide about FDM vs. SLA 3D printers to learn how they compare in terms of print quality, materials, applications, workflow, speed, costs, and more.
In contrast, SLA resin 3D printers create highly isotropic parts. Achieving part isotropy is based on a number of factors that can be tightly controlled by integrating material chemistry with the print process. During printing, resin components form covalent bonds, but layer to layer, the part remains in a semi-reacted “green state.”
While in the green state, the resin retains polymerizable groups that can form bonds across layers, imparting isotropy and water tightness to the part upon final cure. On the molecular level, there is no difference between X, Y, or Z planes. This results in parts with predictable mechanical performance critical for applications like jigs and fixtures, end-use parts, and functional prototyping.
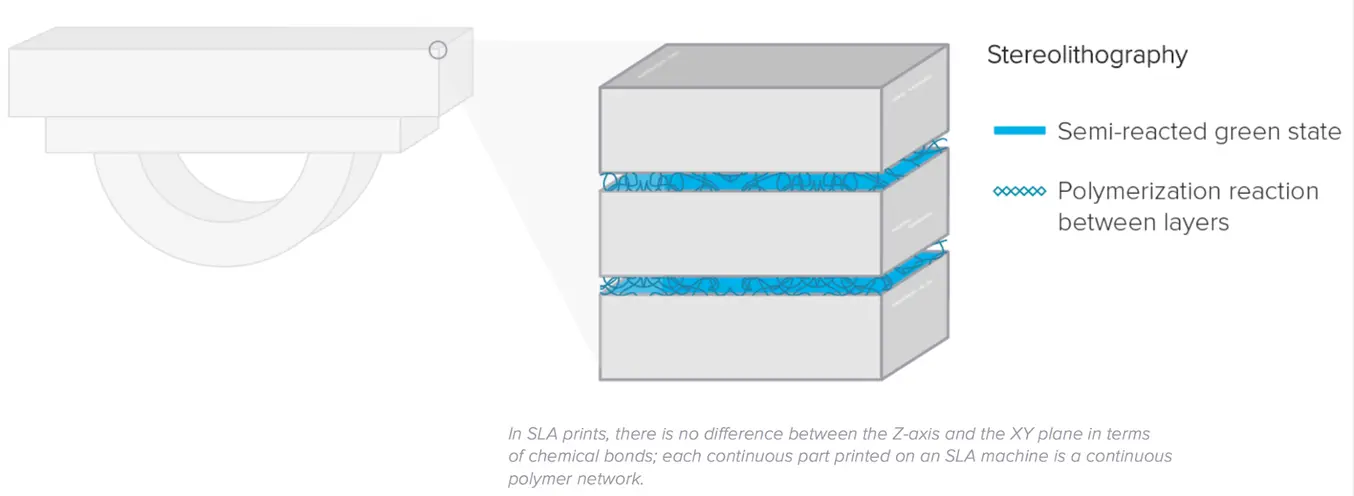
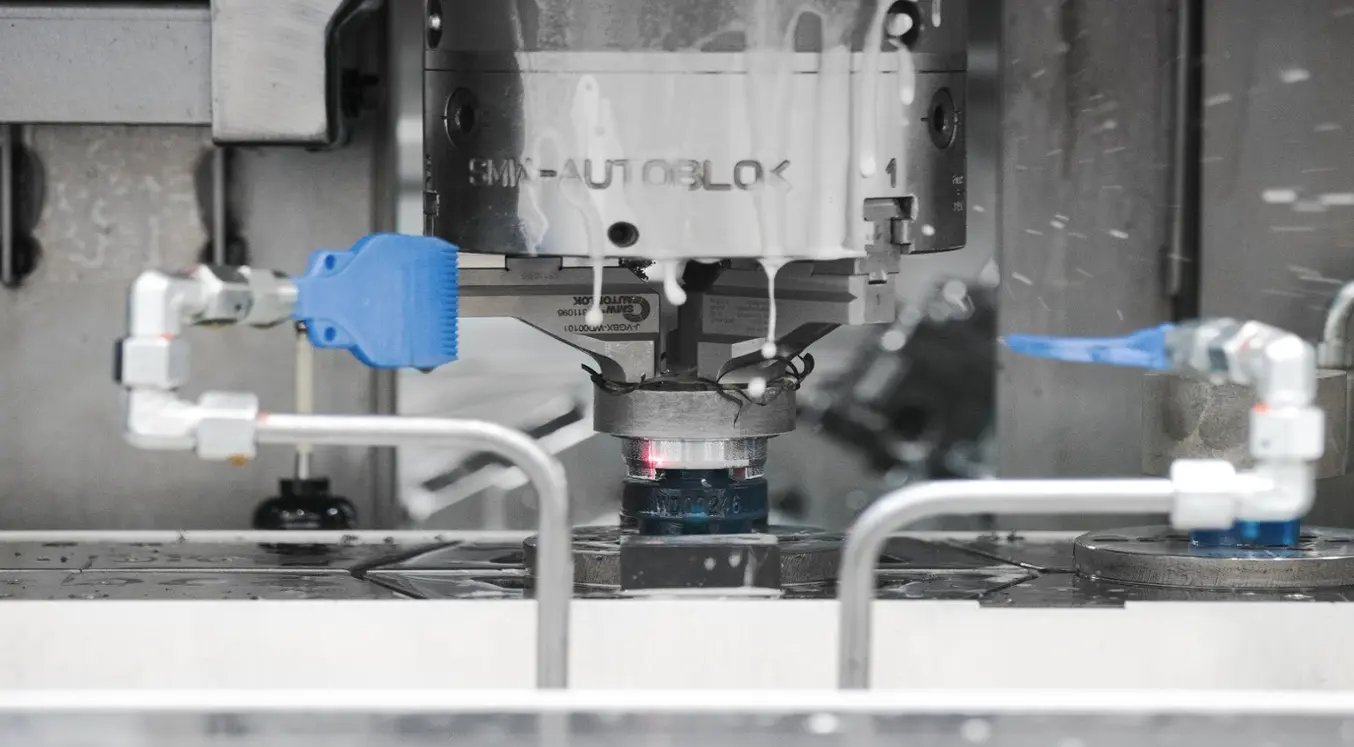
Watertightness
SLA printed objects are continuous, whether producing geometries with solid features or internal channels. This watertightness is important for engineering and manufacturing applications where air or fluid flow must be controlled and predictable. Engineers and designers use the watertightness of SLA printers to solve air and fluid flow challenges for automotive uses, biomedical research, and to validate part designs for consumer products like kitchen appliances.
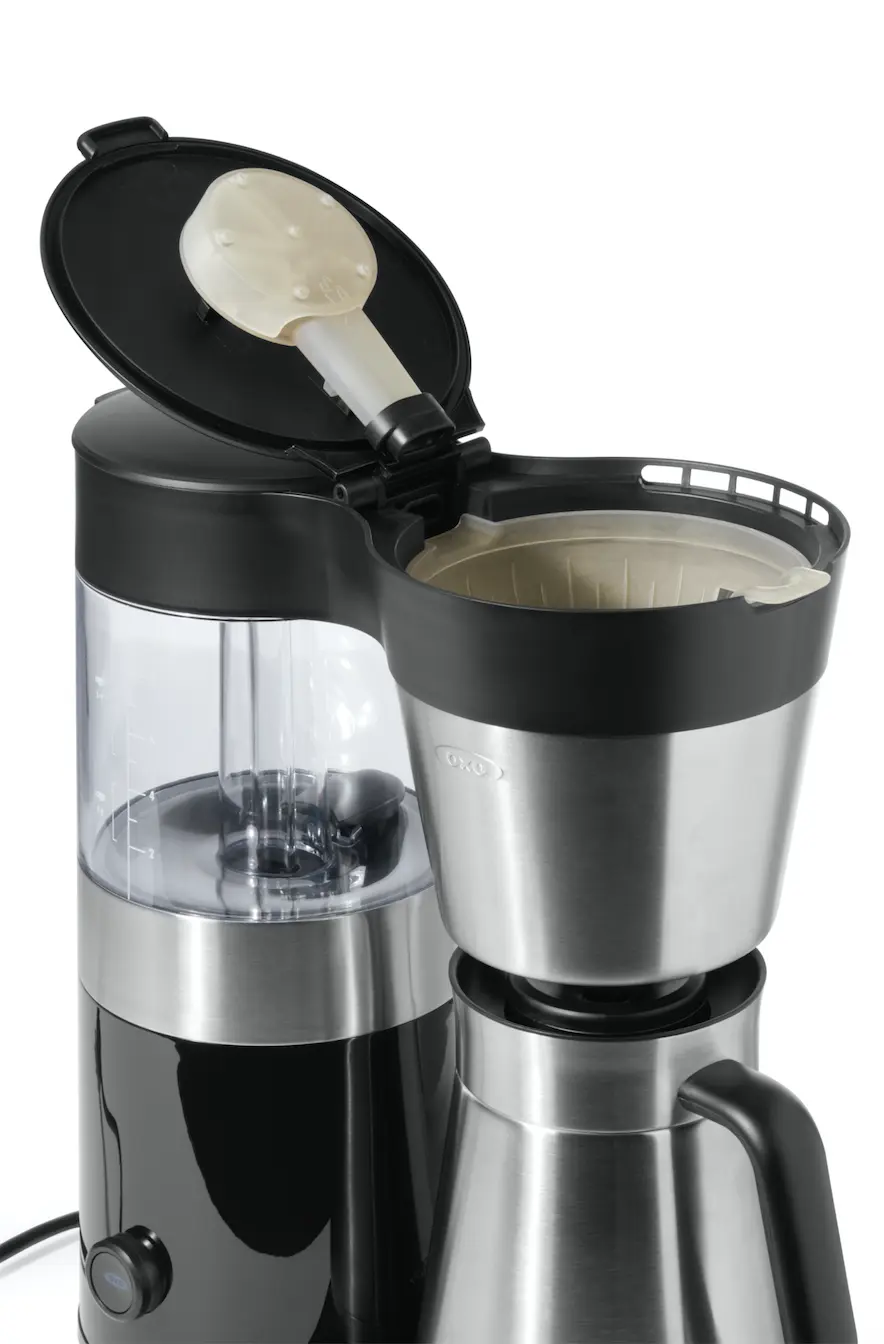
Accuracy and Precision
Industries from dental to manufacturing depend on SLA 3D printing to repeatedly create accurate, precise components. For a print process to produce accurate and precise parts, multiple factors must be tightly controlled.
Compared to machined accuracy, SLA 3D printing is somewhere between standard machining and fine machining. SLA has the highest tolerance of commercially available 3D printing technologies. Learn more about understanding tolerance, accuracy, and precision in 3D printing.
The combination of the heated resin tank and the closed build environment provides almost identical conditions for each print. Better accuracy is also a function of lower printing temperature compared to thermoplastic-based technologies that melt the raw material. Because stereolithography uses light instead of heat, the printing process takes place at close to room temperature, and printed parts don’t suffer from thermal expansion and contraction artifacts.
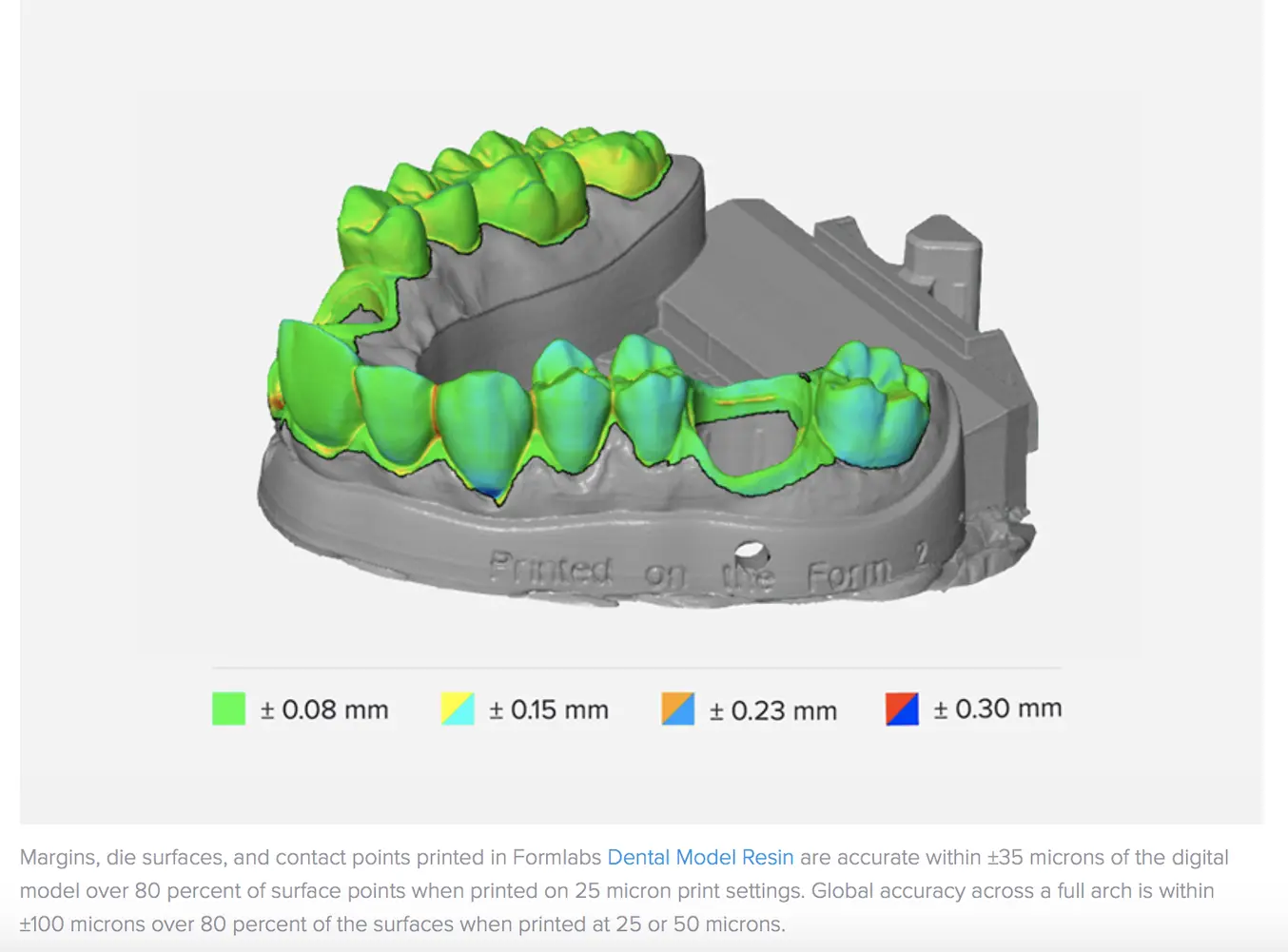
Low Force Stereolithography (LFS) 3D printing houses the optics inside a Light Processing Unit (LPU) that moves in the X-direction. One galvanometer positions the laser beam in the Y direction, then directs it along across a fold mirror and parabolic mirror to deliver a beam that is always perpendicular to the build plane, so it is always moving in a straight line to provide even greater precision and accuracy, and allows for uniformity as hardware scales up to larger sizes, like Formlabs larger format SLA printer Form 3L. The LPU also uses a spatial filter to create a crisp, clean laser spot for greater precision.
The characteristics of individual materials are also important for ensuring a reliable, repeatable print process.
Fine Features and Smooth Surface Finish
SLA printers are considered the gold standard for smooth surface finish, with appearances comparable to traditional manufacturing methods like machining, injection molding, and extrusion.
This surface quality is ideal for applications that require a flawless finish and also helps reduce post-processing time, since parts can easily be sanded, polished, and painted. For example, leading companies like Gillette use SLA 3D printing to create end-use consumer products, like 3D printed razor handles in their Razor Maker platform.
Z-axis layer height is commonly used to define the resolution of a 3D printer. This can be adjusted in between 25 and 300 microns on Formlabs SLA 3D printers, with a trade-off between speed and quality.
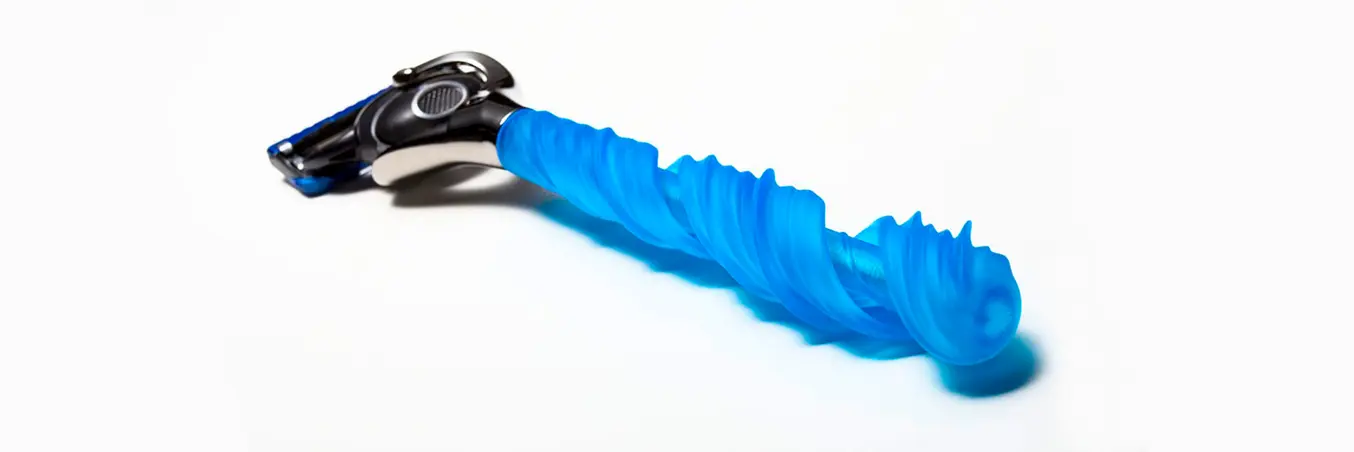
In comparison, FDM and SLS printers typically print Z-axis layers at 100 to 300 microns. However, a part printed at 100 microns on an FDM or SLS printer looks different from a part printed at 100 microns on an SLA printer. SLA prints have a smoother surface finish right out of the printer, because the outermost perimeter walls are straight, and the newly printed layer interacts with the previous layer, smoothing out the staircase effect. FDM prints tend to have clearly visible layers, whereas SLS has a grainy surface from the sintered powder.
The smallest possible detail is also much finer on SLA, given 85 micron laser spot size on the Form 3, in comparison with 350 microns on industrial SLS printers, and 250–800 micron nozzles on FDM machines.
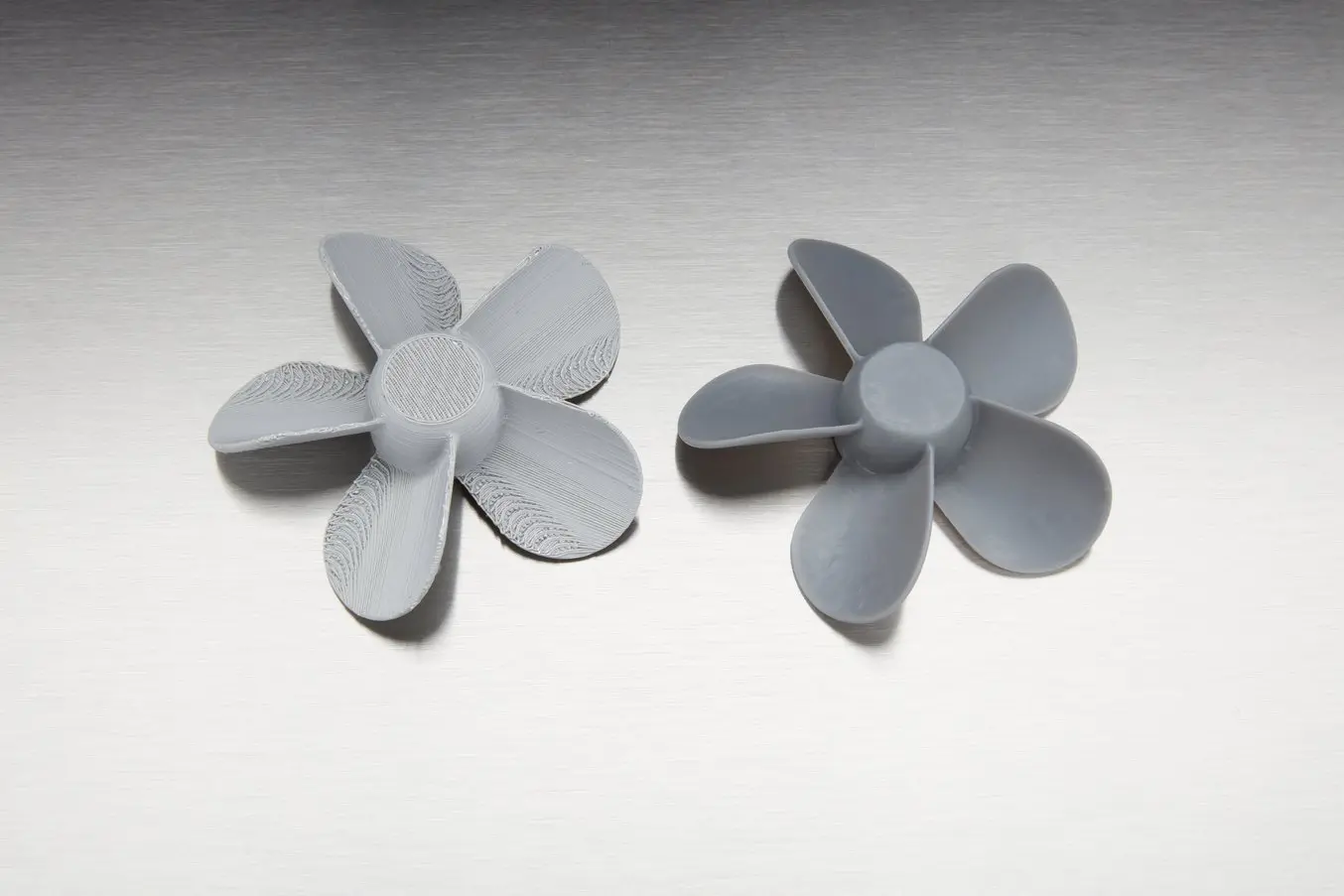
Material Versatility
SLA resins have the benefit of a wide range of formulation configurations: materials can be soft or hard, heavily filled with secondary materials like glass and ceramic, or imbued with mechanical properties like high heat deflection temperature or impact resistance. Material range from industry-specific, like dentures, to those that closely match final materials for prototyping, formulated to withstand extensive testing and perform under stress.
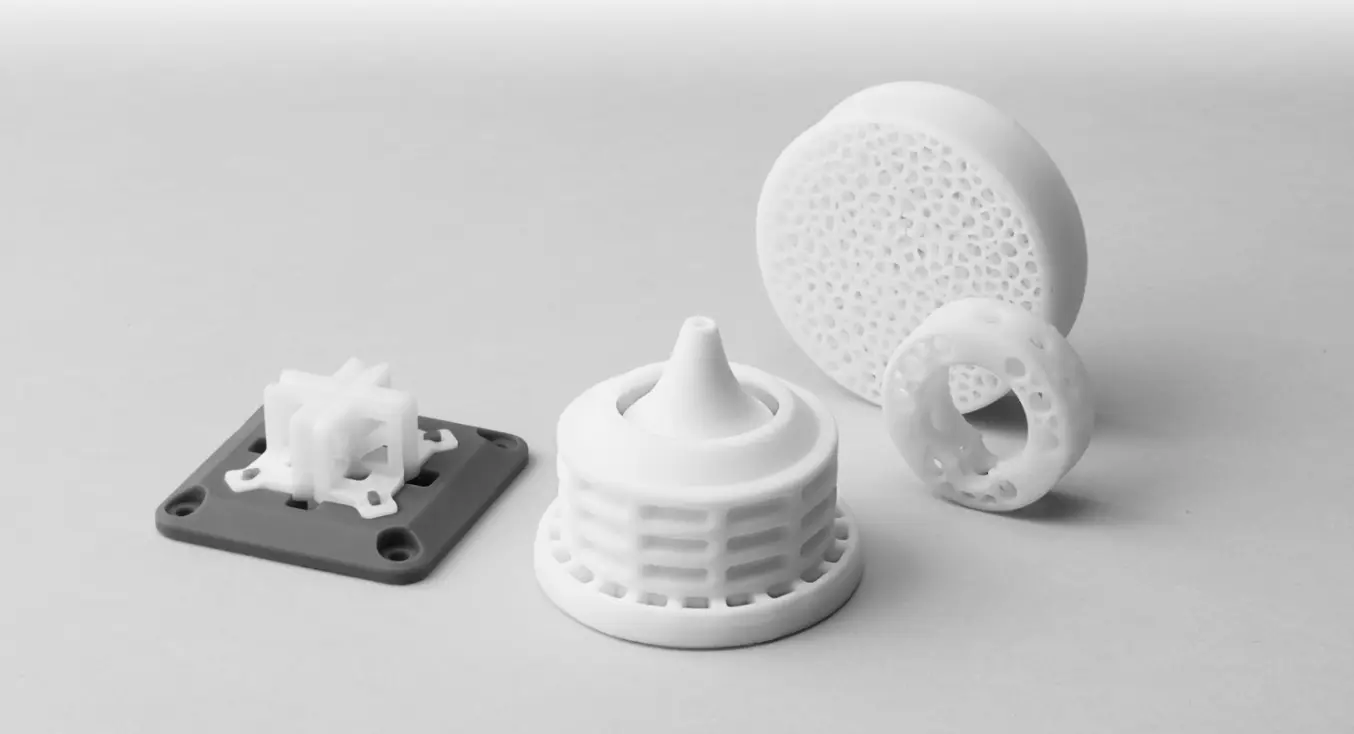
In some cases, its this combination of versatility and functionality that leads to companies to initially bring resin 3D printing in-house. After finding one application solved by a specific functional material, it’s usually not long before more possibilities are uncovered, and the printer becomes a tool for leveraging the diverse capabilities of various materials.
For example, hundreds of engineers in the Design and Prototyping Group at the University of Sheffield Advanced Manufacturing Research Centre (AMRC) rely on open access to a fleet of 12 SLA 3D printers and a variety of engineering materials to support highly diverse research projects with industrial partners like Boeing, Rolls-Royce, BAE Systems, and Airbus. The team used High Temp Resin to 3D print washers, brackets, and a sensor mounting system that needed to withstand the elevated, and leveraged Durable Resin to create intricate custom springy components for a pick and place robot that automates composites manufacturing.
SLA 3D Printing Applications
SLA 3D printing accelerates innovation and supports businesses across a wide range of industries, including engineering, manufacturing, dentistry, healthcare, education, entertainment, jewelry, audiology, and more.
Manufacturing
Rapid prototyping with 3D printing empowers engineers and product designers to turn ideas into realistic proofs of concept, advance these concepts to high-fidelity prototypes that look and work like final products, and guide products through a series of validation stages toward mass production.
Engineering and Product Design
Manufacturers automate production processes and streamline workflows by prototyping tooling and directly 3D printing custom tools, molds, and manufacturing aids at far lower costs and lead times than with traditional manufacturing. This reduces manufacturing costs and defects, increases quality, speeds up assembly, and maximizes labor effectiveness.
Dental
Digital dentistry reduces the risks and uncertainties introduced by human factors, providing higher consistency, accuracy, and precision at every stage of the workflow to improve patient care. 3D printers can produce a range of high-quality custom products and appliances at low unit costs with superior fit and repeatable results.
Education
3D printers are multifunctional tools for immersive learning and advanced research. They can encourage creativity and expose students to professional-level technology while supporting STEAM curricula across science, engineering, art, and design.
Healthcare
Affordable, professional-grade desktop 3D printing helps doctors deliver treatments and devices customized to better serve each unique individual, opening the door to high-impact medical applications while saving organizations significant time and costs from the lab to the operating room.
Entertainment
High definition physical models are widely used in sculpting, character modeling, and prop making. 3D printed parts have starred in stop-motion films, video games, bespoke costumes, and even special effects for blockbuster movies.
Jewelry
Jewelry professionals use CAD and 3D printing to rapidly prototype designs, fit clients, and produce large batches of ready-to-cast pieces. Digital tools allow for the creation of consistent, sharply detailed pieces without the tediousness and variability of wax carving.
Audiology
Hearing specialists and ear mold labs use digital workflows and 3D printing to manufacture higher quality custom ear products more consistently, and at higher volumes for applications like behind-the-ear hearing aids, hearing protection, and custom earplugs and earbuds.
Fast Turnaround Time and Quick Design Changes
Fast turnaround time is a huge advantage to owning a desktop 3D printer. When working with a printing bureau, lead times, communication, and shipping all create delays. With a desktop 3D printer like the Form 3, parts are in-hand within hours, allowing designers and engineers to print multiple parts in one day, helping to iterate faster and drastically reduce product development time and quickly test mechanisms and assemblies avoid costly tool changes.
Cost Savings
Owning a desktop 3D printer results in significant savings over 3D printing service bureaus and traditional machining, as these alternatives rapidly becomes expensive with growing demand and production.
For example, to fulfill tight production deadlines, a process engineer and team at Pankl Racing Systems introduced SLA 3D printing to produce custom jigs and other low-volume parts directly for their manufacturing line. While in-house SLA was initially met with skepticism, it turned out to be an ideal substitute to machining a variety of tools. In one case, it reduced lead time for jigs by 90 percent—from two to three weeks to less than a day—and decreased costs by 80-90 percent.