Additive manufacturing, or 3D printing, lowers costs, saves time, and transcends the limits of fabrication processes for product development. From concept models and functional prototypes in rapid prototyping to jigs, fixtures, or even end-use parts in manufacturing, 3D printing technologies offer versatile solutions in a wide variety of applications.
Over the last few years, high-resolution 3D printers have become more affordable, easier to use, and more reliable. As a result, 3D printing technology is now accessible to more businesses, but choosing between the various competing 3D printing solutions can be difficult.
Which technology is suitable for your particular application? What materials are available? What equipment and training do you need to get started? How about costs and return on investment?
In this article, we’ll take a closer look at the three most established plastic 3D printing processes today: fused deposition modeling (FDM), stereolithography (SLA), and selective laser sintering (SLS).
Trying to decide between FDM and SLA 3D printers? Check out our in-depth FDM vs. SLA comparison.
Fused Deposition Modeling (FDM)
Fused deposition modeling (FDM), also known as fused filament fabrication (FFF), is the most widely used form of 3D printing at the consumer level, fueled by the emergence of hobbyist 3D printers. FDM 3D printers build parts by melting and extruding thermoplastic filament, which a printer nozzle deposits layer by layer in the build area.
FDM works with a range of standard thermoplastics, such as ABS, PLA, and their various blends. The technique is well-suited for basic proof-of-concept models, as well as quick and low-cost prototyping of simple parts, such as parts that might typically be machined.
FDM parts tend to have visible layer lines and might show inaccuracies around complex features. This example was printed on a Stratasys uPrint industrial FDM 3D printer with soluble supports (machine starting at $15,900).
FDM has the lowest resolution and accuracy when compared to SLA or SLS and is not the best option for printing complex designs or parts with intricate features. Higher-quality finishes may be obtained through chemical and mechanical polishing processes. Industrial FDM 3D printers use soluble supports to mitigate some of these issues and offer a wider range of engineering thermoplastics, but they also come at a steep price.
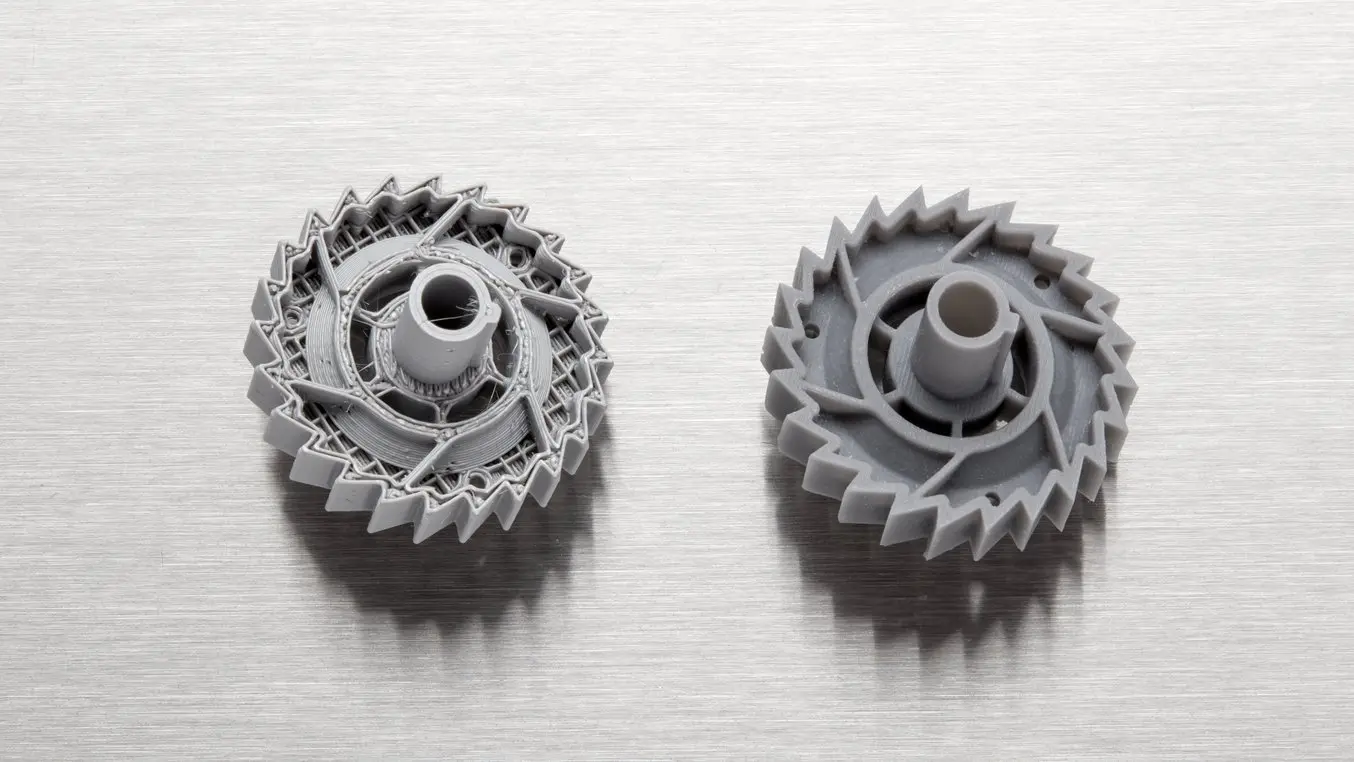
Stereolithography (SLA)
Stereolithography was the world’s first 3D printing technology, invented in the 1980s, and is still one of the most popular technologies for professionals. SLA resin 3D printers use a laser to cure liquid resin into hardened plastic in a process called photopolymerization.
SLA parts have the highest resolution and accuracy, the clearest details, and the smoothest surface finish of all plastic 3D printing technologies, but the main benefit of SLA lies in its versatility. Material manufacturers have created innovative SLA photopolymer resin formulations with a wide range of optical, mechanical, and thermal properties to match those of standard, engineering, and industrial thermoplastics.
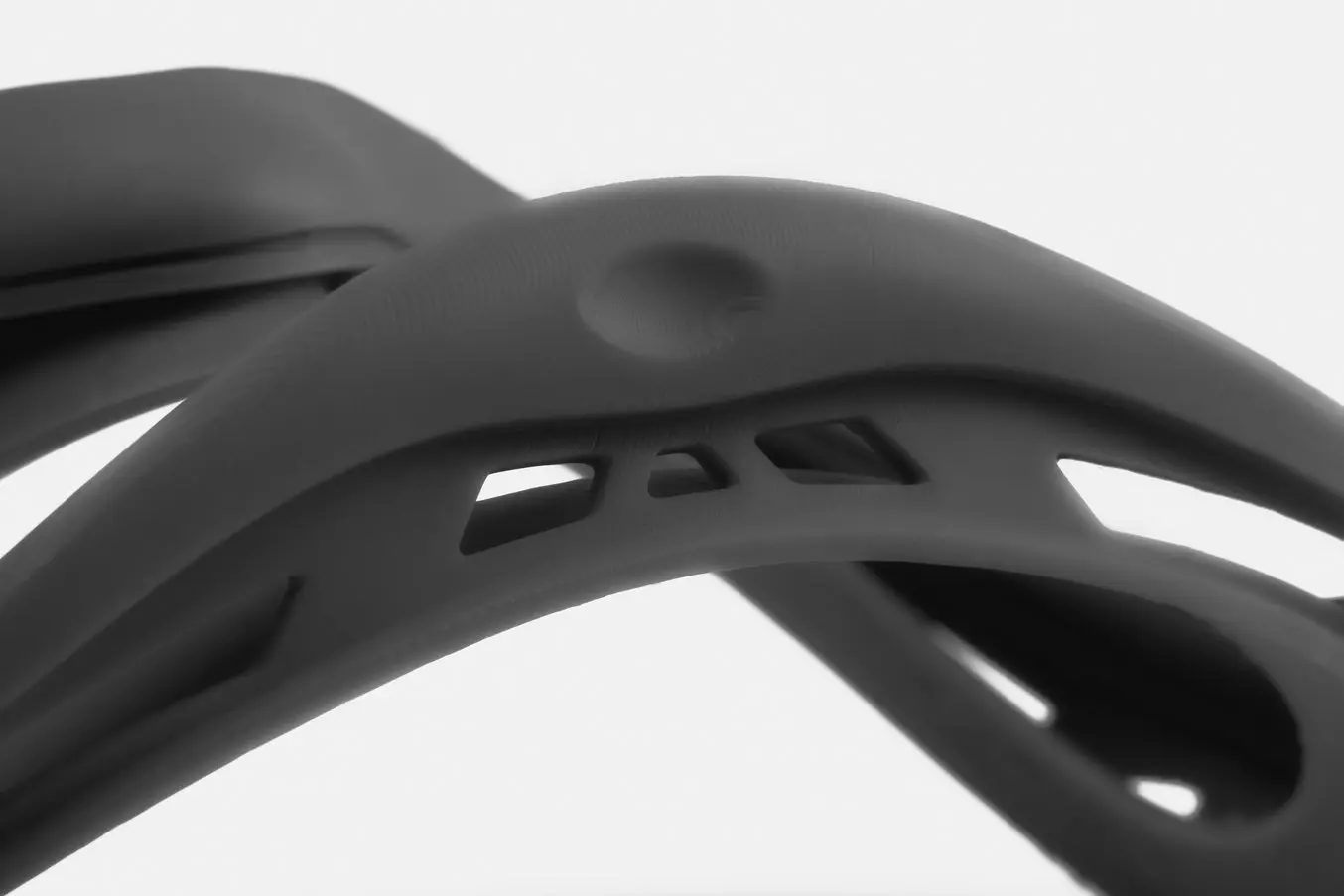
SLA is a great option for highly detailed prototypes requiring tight tolerances and smooth surfaces, such as molds, patterns, and functional parts. SLA is widely used in a range of industries from engineering and product design to manufacturing, dentistry, jewelry, model making, and education.
Selective Laser Sintering (SLS)
Selective laser sintering is the most common additive manufacturing technology for industrial applications, trusted by engineers and manufacturers across different industries for its ability to produce strong, functional parts.
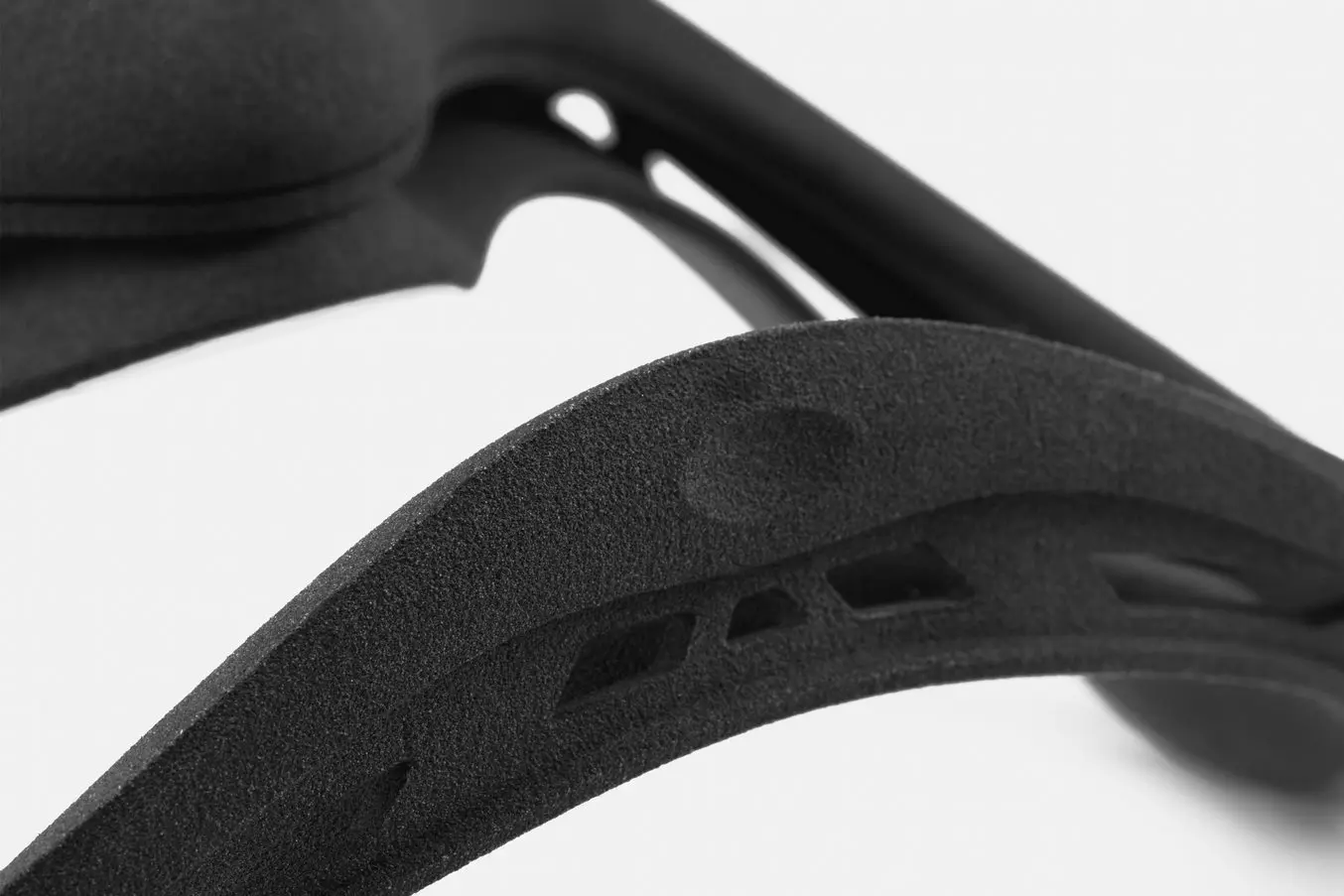
The most common material for selective laser sintering is nylon, a popular engineering thermoplastic with excellent mechanical properties. Nylon is lightweight, strong, and flexible, as well as stable against impact, chemicals, heat, UV light, water, and dirt.
The combination of low cost per part, high productivity, and established materials make SLS a popular choice among engineers for functional prototyping, and a cost-effective alternative to injection molding for limited-run or bridge manufacturing.
Compare FDM, SLA, and SLS 3D Printing Technologies
Each 3D printing technology has its own strengths, weaknesses, and requirements, and is suitable for different applications and businesses. The following table summarizes some key characteristics and considerations.
FUSED DEPOSITION MODELING (FDM) | STEREOLITHOGRAPHY (SLA) | SELECTIVE LASER SINTERING (SLS) | |
---|---|---|---|
Resolution | ★★☆☆☆ | ★★★★★ | ★★★★☆ |
Accuracy | ★★★★☆ | ★★★★★ | ★★★★★ |
Surface Finish | ★★☆☆☆ | ★★★★★ | ★★★★☆ |
Throughput | ★★★★☆ | ★★★★☆ | ★★★★★ |
Complex Designs | ★★★☆☆ | ★★★★☆ | ★★★★★ |
Ease of Use | ★★★★★ | ★★★★★ | ★★★★☆ |
Pros | Fast Low-cost consumer machines and materials |
Great value High accuracy Smooth surface finish Range of functional applications |
Strong functional parts Design freedom No need for support structures |
Cons | Low accuracy Low details Limited design compatibility |
Sensitive to long exposure to UV light | Rough surface finish Limited material options |
Applications | Low-cost rapid prototyping Basic proof-of-concept models |
Functional prototyping Patterns, molds, and tooling Dental applications Jewelry prototyping and casting Modelmaking |
Functional prototyping Short-run, bridge, or custom manufacturing |
Print Volume | Up to 300 x 300 x 600 mm (desktop and benchtop 3D printers) | Up to 300 x 335 x 200 mm (desktop and benchtop 3D printers) | Up to 165 x 165 x 300 mm (benchtop industrial 3D printers) |
Materials | Standard thermoplastics, such as ABS, PLA, and their various blends. | Varieties of resin (thermosetting plastics). Standard, engineering (ABS-like, PP-like, flexible, heat-resistant), castable, dental, and medical (biocompatible). | Engineering thermoplastics. Nylon 11, Nylon 12, and their composites. |
Training | Minor training on build setup, machine operation, and finishing; moderate training on maintenance. | Plug and play. Minor training on build setup, maintenance, machine operation, and finishing. | Moderate training on build setup, maintenance, machine operation, and finishing. |
Facility Requirements | Air-conditioned environment or preferably custom ventilation for desktop machines. | Desktop machines are suitable for an office environment. | Workshop environment with moderate space requirements for benchtop systems. |
Ancillary Equipment | Support removal system for machines with soluble supports (optionally automated), finishing tools. | Post-curing station, washing station (optionally automated), finishing tools. | Post-processing station for part cleaning and material recovery. |
3D Printing Costs and Return on Investment With FDM, SLA, and SLS Technologies
Ultimately, you should choose the technology that makes the most sense for your business. Prices have dropped significantly in recent years, and today, all three technologies are available in compact, affordable systems.
Calculating 3D printing costs does not end with upfront equipment costs. 3D printing material and labor costs have a significant influence on cost per part, depending on the application and your production needs.
Here’s a detailed breakdown by technology:
FUSED DEPOSITION MODELING (FDM) | STEREOLITHOGRAPHY (SLA) | SELECTIVE LASER SINTERING (SLS) | |
---|---|---|---|
Equipment Costs | Budget printers and 3D printer kits start at a few hundred dollars. Higher quality mid-range desktop printers start around $2,000, and industrial systems are available from $15,000. | Professional desktop printers start at $3,500, large-format benchtop printers at $11,000, and large-scale industrial machines are available from $80,000. | Benchtop industrial systems start at $18,500, and traditional industrial printers are available from $100,000. |
Material Costs | $50-$150/kg for most standard and engineering filaments, and $100-200/kg for support materials. | $149-$200/L for most standard and engineering resins. | $100/kg for nylon. SLS requires no support structures, and unfused powder can be reused, which lowers material costs. |
Labor Needs | Manual support removal (can be mostly automated for industrial systems with soluble supports). Lengthy post-processing is required for a high-quality finish. | Washing and post-curing (both can be mostly automated). Simple post-processing to remove support marks. | Simple cleaning to remove excess powder. |
Learn More About 3D Printing
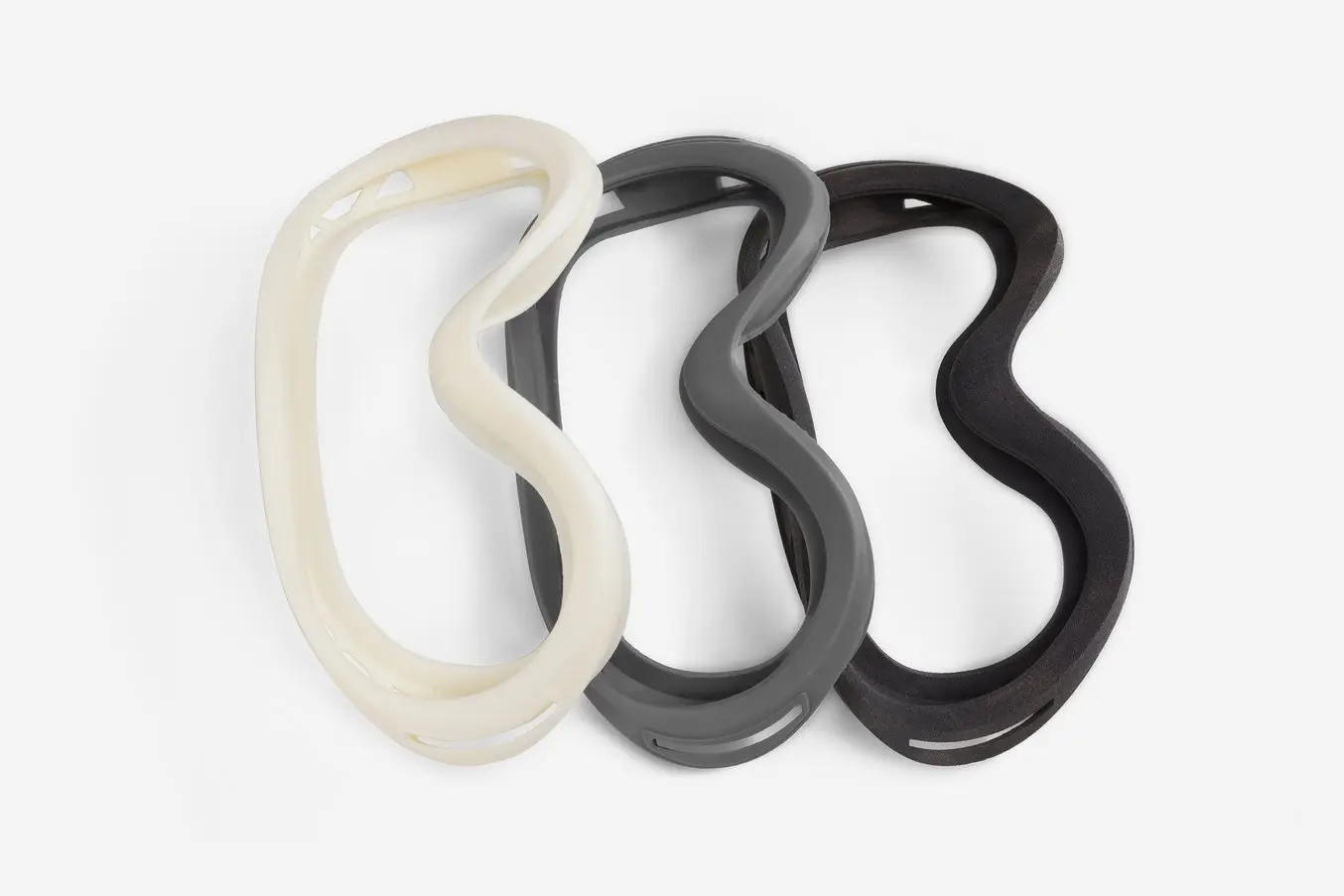
We hope this article has helped you focus your search for the best 3D printing technology for your application.
Explore our additional resources to master the intricacies of 3D printing, and dive deeper into each technology to learn more about specific 3D printing systems.