Bioinks are the “filament” of bioprinters. Have you ever wondered what’s inside them or how they work? Let us give you the tour.
Background
Bioink is the material used to produce engineered (artificial) live tissue using 3D printing technology. It can be composed only of cells, but in most cases, an additional carrier material that envelops the cells is also added. This carrier material is usually a biopolymer gel, which acts as a 3D molecular scaffold. Cells attach to this gel, and this enables them to spread, grow and proliferate.
Importantly, the gel can also provide protection to the cells during the printing process. Its importance is so high that the term “bioink” is often commonly used to describe the carrier material alone, irrespective of the cells that may grow on it.
Bioinks Are Gels, Not Filaments!
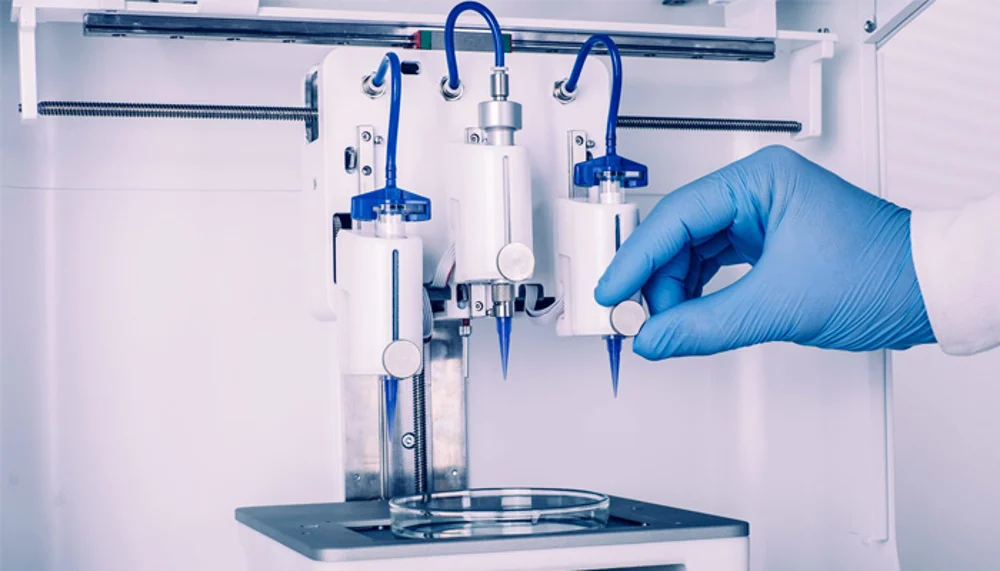
An important characteristic of the biopolymer (or mixture of biopolymers) in bioink is its capability to retain water, making it a hydrogel. This hydrogel takes a predominant role in the mechanical stability of engineered tissue, which will influence the 3D fidelity of the object being bioprinted.
One of the key properties of any gel is the ability to shift between liquid-like and solid-like states when the necessary conditions are applied. How we can drive this “sol-gel” transition depends on the gel system being considered. In turn, this will dictate the exact bioprinting technique used.
Here we take a closer look at the polymer formulation behind bioinks in extrusion-based systems, which are the most common.
A Delicate Balance
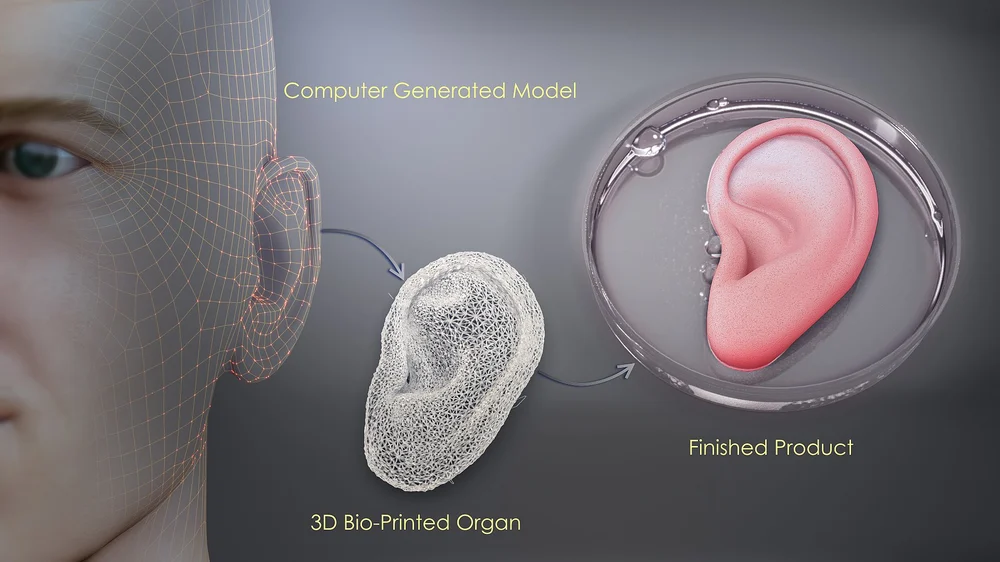
To fabricate a part in an FDM printer, we use temperature to exploit phase transitions in thermoplastics. We drive them from a solid to a molten state as they cross the extruder. And then they become solid again as they cool down. Likewise, bioinks must go through some form of phase transition that enables their accurate distribution in space.
Both thermoplastics and bioinks are made of polymers, but polymers can be very very very (did we mention “very” yet?) different. The polymers in bioinks must be able to form a gel of a fairly sturdy consistency as this will enable a high printing fidelity.
However, the polymers in the gel cannot form such a tight and stiff network to literally imprison the cells. The network must reach a sweet spot in its material properties: it should enable printing with high fidelity yet be flexible enough to accommodate flow and diffusion of nutrients and movement of cells.
To achieve this, one needs to control either chemical or physical factors that enable the hydrogels to undergo these phase transitions. In addition, this should occur while simultaneously keeping cells alive during the printing process. As you will see, this balance of factors is more difficult than one might think.
Melting a Bioink
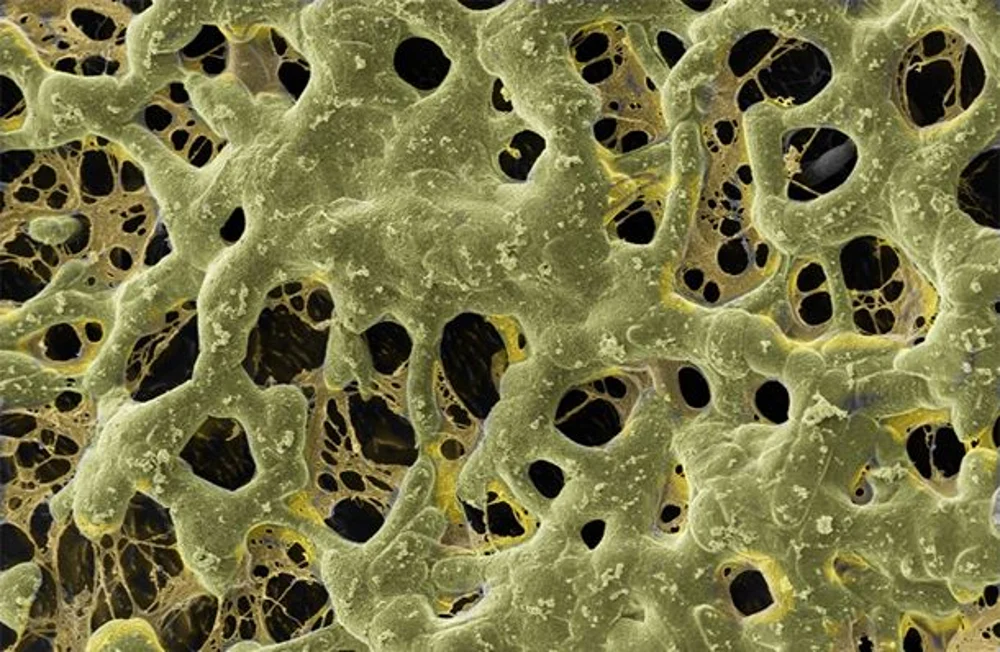
One of the possibilities to control gelation is through temperature. This is true for gelatin, for example, which is the base material of some bioinks.
Like in FDM, the extruder can include a heating element that slightly increases the temperature. The key is to partially melt the polymer, allowing it to pass through the nozzle.
Naturally, the temperature experienced by the bioink at the extruder cannot be too high otherwise the cells will be cooked. This means that not all polymers will be adequate to act as bioinks for bioprinters that employ this type of extrusion system.
Thinning a Bioink
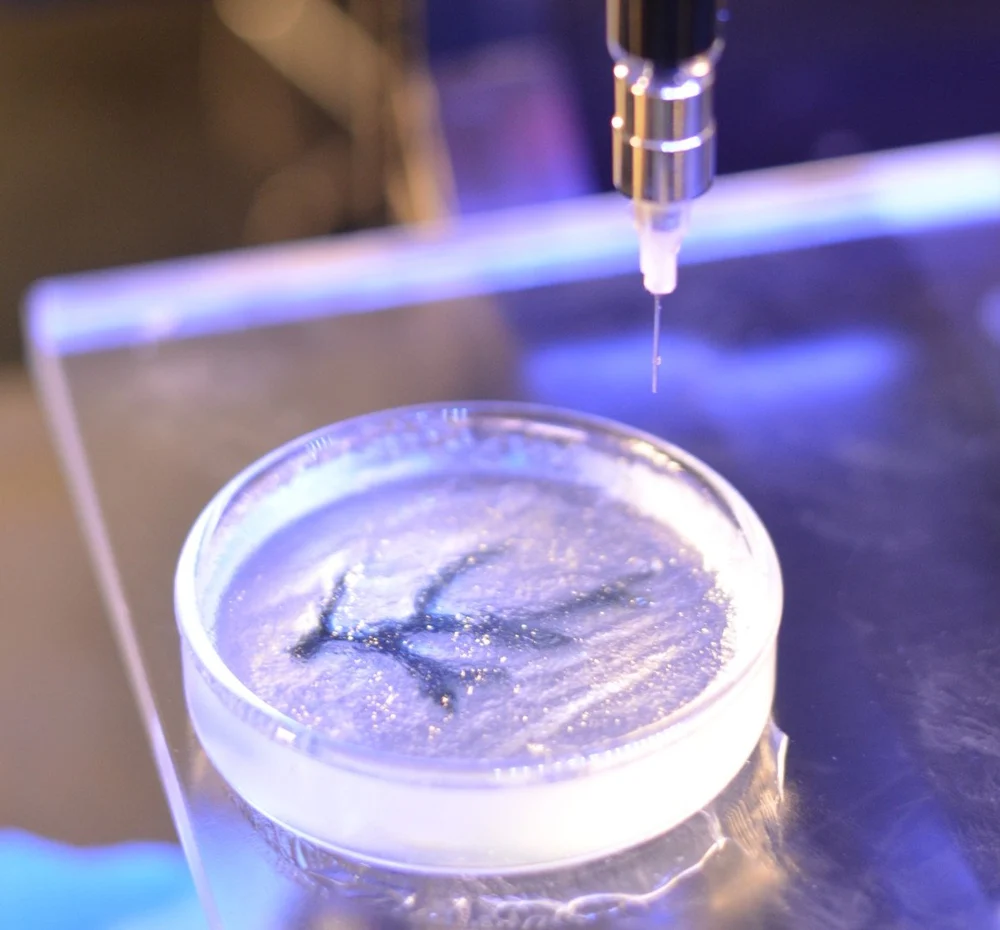
Other forms of inducing the so-called sol-gel transition rely on a phenomenon known as “shear-thinning”. Some gels become more liquid-like when they experience shear stress.
One example is natural yogurt, which has the consistency of a sturdy gel until you decide to mix it. After a few turns of a spoon, all that shear caused by the movement of the spoon renders the yogurt more fluid-like. Not all gels behave like this, however. Try to do the same thing with gelatin to see if it will work…
Shear Printing
Bioinks designed to experience shear-thinning are accelerated through a narrow nozzle thanks to the mounting pressure at the bioink reservoir. The shear is caused by the rubbing of the gel against the walls of the needle-like nozzle. The increased fluidity in the gel allows it to become printable.
As the bioink arrives at the print bed, it stops experiencing significant motion. In this way, shear stress drops abruptly, which leads to a rapid gelation. Since this method does not require an increase in temperature, it’s typically the one that is the most cell-friendly.
Cell Health
However, the shear forces experienced by materials as they’re pushed through the extrusion system are often not negligible to the health of cells. Interactions like cells bumping up against a wall are often underappreciated when discussed at the level of the cell.
Here the viscoelastic properties of the gel help to reduce the shear forces that are associated with the friction experienced by cells as they travel through narrow channels, causing them to deform. Gel intervenes the same way dampeners help to cushion your car ride on a bumpy road. It helps to dissipate forms of kinetic energy that are potentially damaging.
Other forms of inducing the so-called sol-gel transition rely on a phenomenon known as “shear-thinning”. Some gels become more liquid-like when they experience shear stress.
One example is natural yogurt, which has the consistency of a sturdy gel until you decide to mix it. After a few turns of a spoon, all that shear caused by the movement of the spoon renders the yogurt more fluid-like. Not all gels behave like this, however. Try to do the same thing with gelatin to see if it will work…
Shear Printing
Bioinks designed to experience shear-thinning are accelerated through a narrow nozzle thanks to the mounting pressure at the bioink reservoir. The shear is caused by the rubbing of the gel against the walls of the needle-like nozzle. The increased fluidity in the gel allows it to become printable.
As the bioink arrives at the print bed, it stops experiencing significant motion. In this way, shear stress drops abruptly, which leads to a rapid gelation. Since this method does not require an increase in temperature, it’s typically the one that is the most cell-friendly.
Cell Health
However, the shear forces experienced by materials as they’re pushed through the extrusion system are often not negligible to the health of cells. Interactions like cells bumping up against a wall are often underappreciated when discussed at the level of the cell.
Here the viscoelastic properties of the gel help to reduce the shear forces that are associated with the friction experienced by cells as they travel through narrow channels, causing them to deform. Gel intervenes the same way dampeners help to cushion your car ride on a bumpy road. It helps to dissipate forms of kinetic energy that are potentially damaging.
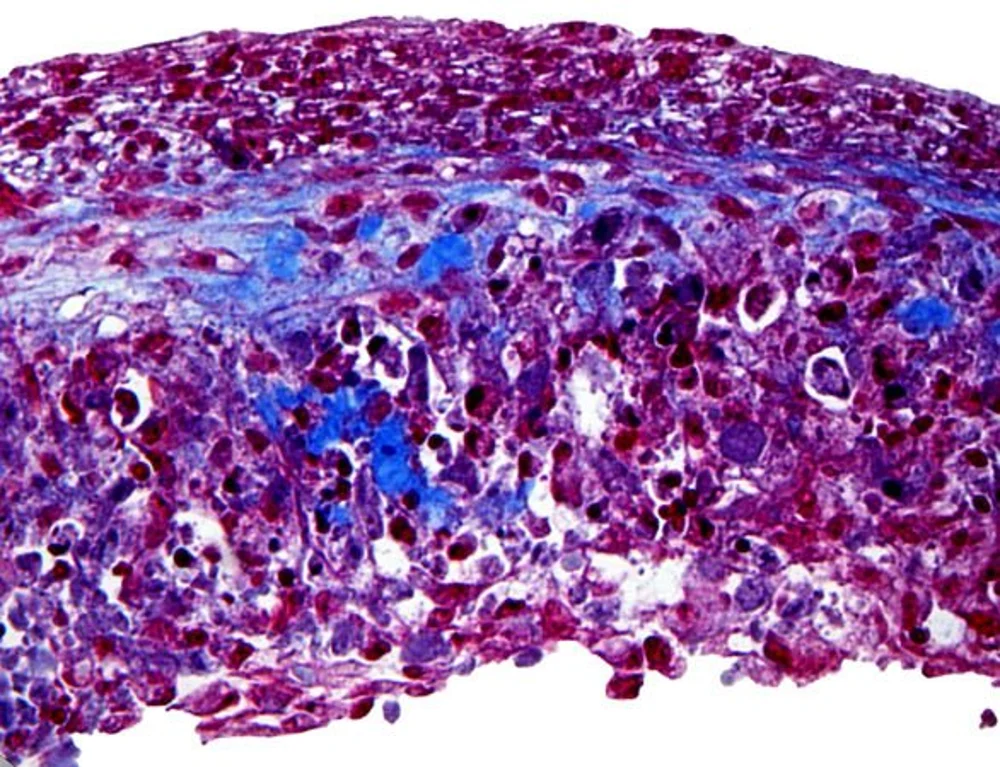
To safely deliver cells across the nozzle and into the printing bed is only one of the tasks of bioink. Another is to serve as the cells’ food source, or to at least be amenable to be degraded by them.
As cells grow and proliferate, they typically modify their environment. They take in substances found outside, metabolize them, and then release different ones.
In fact, many of the substances that cells produce and eject are not necessarily the equivalent of toxic material they need to get rid of. Instead, they also take care of their local environment. They also recycle it and produce a matrix of substances to surround them and increase their chances of survival.
This extracellular matrix is the stuff that cells need, and they often produce it by themselves. Thus, a good artificial tissue is one where all artificial elements have been replaced by the natural ones. This enables one of the key applications of 3D bioprinting, which is the implantation of the artificially produced organs or body parts.
List of Ingredients
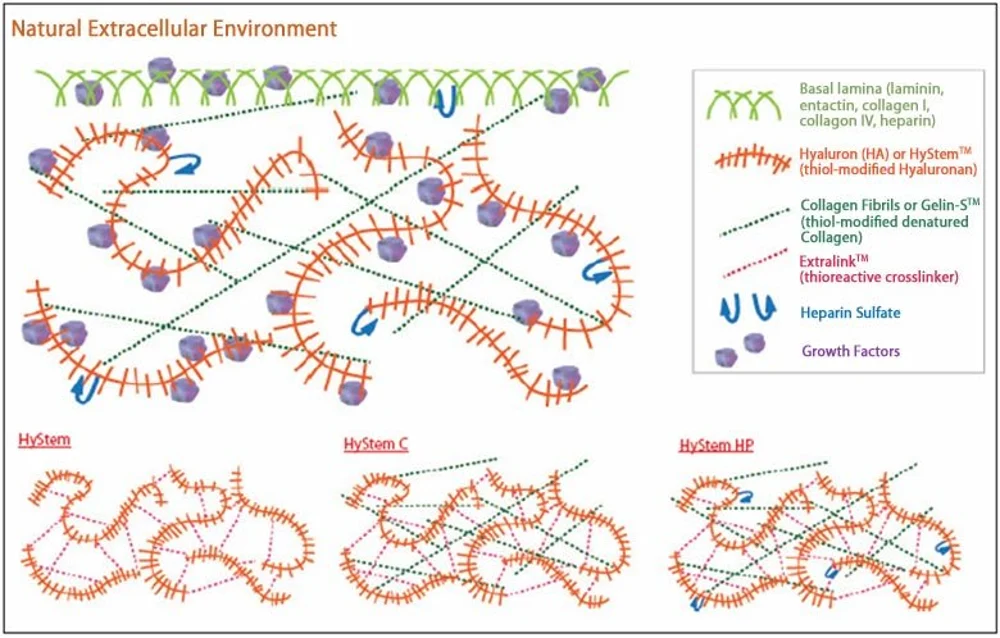
Often bioinks rely on a combination of several polymers to achieve some sort of middle ground where chemical, physical, and biological constraints are respected. Here’s a small list of the polymers you may find inside a bioink:
- Agarose is a marine polysaccharide obtained from seaweed. It’s been a long-time favorite due to its commendable biocompatibility and gelation properties. But agarose doesn’t provide cells with the necessary anchoring points that they require to move about. Therefore it usually appears in bioinks mixed together with other polymers.
- Alginate is another vegetable marine biopolymer that can entrap water and nutrients from the surrounding medium. This property is ideal for 3D bioprinting since limited diffusion of nutrients is one of the biggest hurdles that cells face inside dense structures, such as living tissues.
- Collagen, commonly known as gelatin, is another common component of bioinks. You can find collagen in great quantities inside animal muscles and tendons and this is not by chance. Collagen has a stiff fibrous molecular structure, has great viscoelastic properties, enhances the mechanical stability of tissues where it is found, and cells love to stick to it. These days you can find synthetic variants of collagen that impart much of the properties of the natural stuff without having to kill animals for it.
- Hyaluronic acid is a ubiquitous molecule in the extracellular space of animal cells and an important component of articular cartilage. It’s a water-loving molecule that, after its discovery, was knick-named the “goo molecule”. Although its consistency is not always ideal in 3D bioprinting, many cells simply love it. This is why artificial variants of hyaluronic acid with enhanced mechanical properties can be found in bioinks.
The list of components of bioinks is quite a match for the materials that you can use in FDM printing. From proteins involved in blood-clotting, to cellulose or silk, you can also add purely synthetic materials such as polyethylene glycol (PEG), Pluronic, or different methacrylates. As the R&D efforts in 3D bioprinting develop and the interest in personalized medicine makes further steps, we can expect the market of bioinks to expand enormously over the next few years.
Source: https://all3dp.com/2/for-ricardo-what-is-bioink-simply-explained/